Overview
The production of acoustic waves and detection of the resultant echoes is based upon the pulse-echo-principle.
The acoustic wave is generated by application of an electrical current to a piezoelectric crystal, inducing mechanical stress and deformation (the converse piezoelectric effect) which in turn generates pressure waves (acoustic waves).
After the acoustic wave has been generated the crystal switches to detection mode and awaits returning acoustic echoes.
This cycle is repeated several million times per second.
In practice, 99 per cent of the time is spent detecting echoes.
Additional information
Acoustic wave propagation through media
Once the acoustic wave is generated, it travels through the media with which it comes into contact. The speed at which these acoustic waves travel through the sample is influenced by the density and stiffness of the materials within the sample. Acoustic waves travel faster through stiffer materials.
Acoustic impedance
Acoustic impedance refers to the resistance to the passage of ultrasound waves. The greater the acoustic impedance, the more resistant the tissue is to the passage of ultrasound waves.
Reflection at boundaries
When the acoustic wave encounters a boundary between two different media, some of the sound wave continues to propagate through the tissue, while some of the wave bounces back toward the source as an echo (reflection). The angle of incidence is identical to the angle of reflection.
Refraction of acoustic waves
When the acoustic wave travels through a different medium, it bends from its original path (refraction). The angle of incidence will differ from the angle of transmission. The extent of deflection depends on the difference in stiffness between the two tissues.
Scattering of acoustic waves
Scattering occurs when acoustic waves encounter a medium with a nonhomogeneous surface. A small portion of the acoustic wave is scattered in random directions, while most of the original wave continues along its initial path. Reflection of the acoustic waves happens at a boundary or interface between tissues with different acoustic impedance.
Attenuation of acoustic waves
When traveling through a medium, the intensity and amplitude of the acoustic wave are reduced (attenuated). As a result, echoes from deeper structures are weaker compared to echoes from superficial areas. The primary source of attenuation in soft tissue is absorption, which is the conversion of acoustic energy into heat. However, reflection, refraction, and scatter also contribute to attenuation.
Acoustic wave velocity in human tissue
In human tissue at body temperature, acoustic waves travel at an average velocity of approximately 1540 m/s.
Reflection at tissues with different acoustic impedance
The strongest reflections of echoes back to the probe occur at the interfaces of tissues with the greatest differences in acoustic impedance. For instance, there is a large difference between the acoustic impedance of air and soft tissue, as well as between the acoustic impedance of bone and soft tissue. These interfaces result in very strong echoes, producing what are termed hyperechoic images.
Hypoechoic images
In contrast, the differences in acoustic impedance between various types of soft tissue, such as blood, muscle, and fat, are very small. This results in hypoechoic images, where the echoes are weaker and less distinct.
Frequency range for diagnostic ultrasound
Diagnostic ultrasound typically uses frequencies between 2 and 20 MHz. The choice of frequency depends on the depth of the structures to be imaged and the spatial resolution required.
Frequency and tissue penetration
Lower frequencies can penetrate deeper into tissue but produce images with poorer resolution. Conversely, higher frequencies provide better spatial resolution but have reduced tissue penetration.
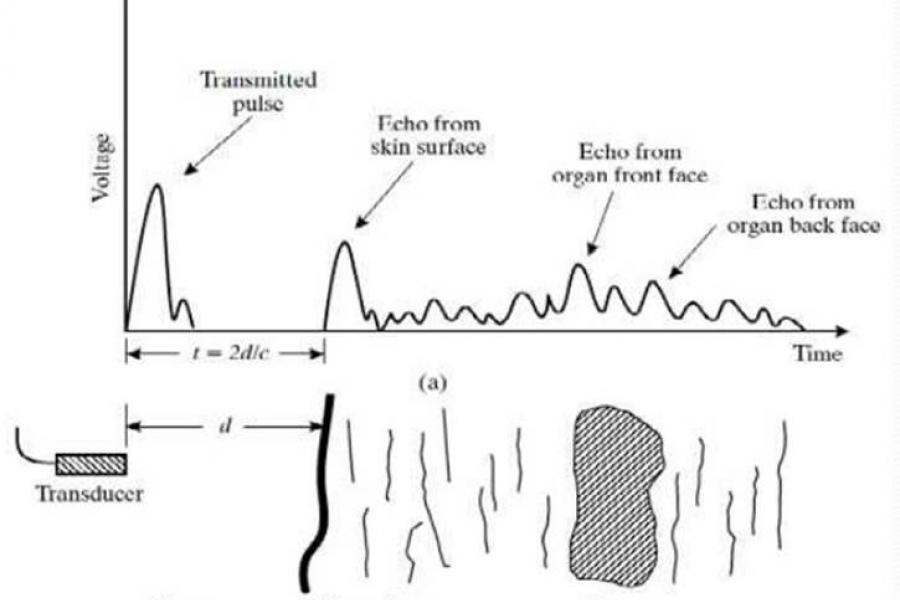
Examples
Instrumentation
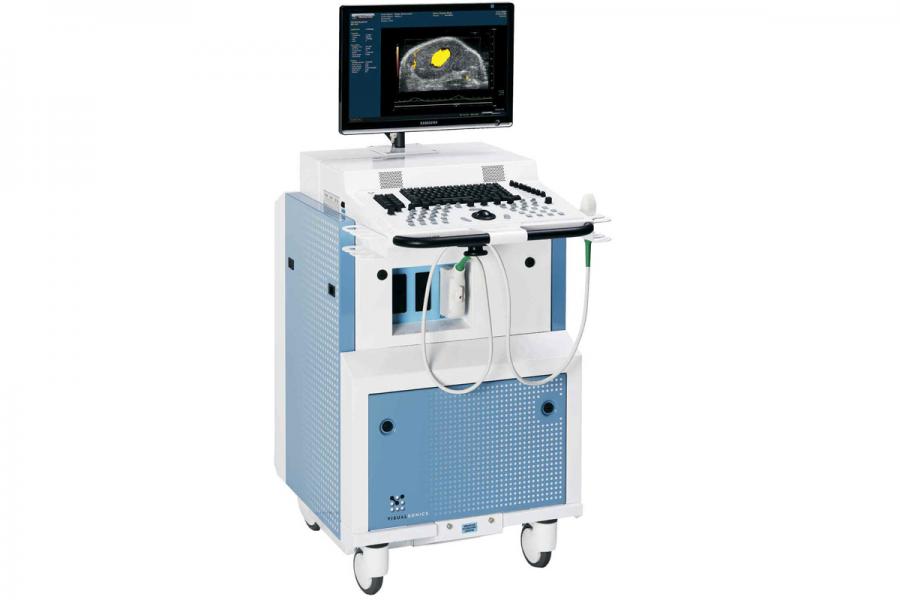
VisualSonics Vevo 2100
Ultrasound imaging is performed using a VisualSonics Vevo 2100 imaging system equipped with 24, 38, 55 and 70 MHz transducers.
Additional information
A number of operational modes are available, including:
M-mode
Motion mode, single line acquisition allowing high-temporal resolution for analysis of cardiovascular function.
Anatomical M-mode
Allows the user to steer the sample volume to any angle rather than having sample volume in a strict vertical position.
PW Doppler mode
Pulsed-wave Doppler acquisition for blood flow velocity and waveform measurements.
PW Tissue Doppler mode
Displays lower velocity signals such as those from the left ventricular (LV) muscle for assessing diastolic dysfunction and other cardiac conditions.
Colour Doppler mode
Two-dimensional and colour acquisition for blood flow velocity analysis.
3-D mode
Volumetric acquisition of tumours and other structures for quantification and assessment.
Applications
Applications include:
- Abdominal imaging
- Cardiology
- Cardiovascular imaging
- Embryology
- Epidermal imaging
- Ocular imaging
- Reproductive system imaging
- Tumour imaging
- Vascular imaging
Resources
Application notes
The materials listed here are available for investigators by request.
Cardiac measurements
Diastolic dysfunction
Nephrology
Pulmonary hypertension
Reproductive and developmental biology
Tumour angiogenesis
Tumour vascularity
Manuals
The materials listed here are available for investigators by request.
VEVO 2100 operators manual
VEVO 210 Quick start guide
Training videos
The materials listed here are available for investigators by request.
Ransthoracic eccocardiography in mice
Contact us
Small Animal and Material Imaging Core Facility (SAMICF)
23 Basic Medical Sciences Building
745 Bannatyne Avenue
University of Manitoba
Winnipeg, MB R3E 0J9 Canada